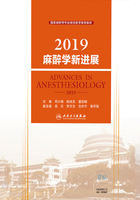
14 血-脑屏障和神经鞘脂在脓毒症脑病中枢炎症中的作用及机制剖析
脓毒症是全球发病率和死亡率的主要原因。2016年发布SSC指南进一步完善了脓毒症的定义,将其称之为“因宿主对感染反应失调而导致的危及生命的器官功能障碍”。如果抑制炎症反应和恢复器官灌注的努力失败,将导致脓毒性休克和多器官功能障碍。血-脑屏障(blood-brain barrier,BBB)的破坏会导致意识的显著改变和神经认知能力的降低。脓毒症引起的脑功能障碍,在临床上表现为从急性意识混乱状态开始到最终昏迷等一系列连续性神经精神障碍。排除药物诱导和其他代谢病因,这种综合征被称为“脓毒症脑病(sepsis-associated encephalopathy,SAE)”,这是ICU内最常见的脑病形式。脓毒症脑病与谵妄有一些相同特征,包括发病迅速和认知明显恶化,但谵妄是SAE的一种类型,包括意识过度兴奋和抑制等类型。在既往文献中,SAE多被理解为存在脓毒症,但不存在CNS感染和其他形式脑病的情况下出现的弥漫性脑功能障碍。其表现通常包括睡眠-觉醒周期紊乱、意识受损、轻度认知功能障碍、明显的谵妄和昏迷等。
SAE是对公众健康的巨大威胁,它对患者短期发病率、住院时间延长、长期认知障碍都有明显影响,并对医疗保健系统造成巨大的经济负担。SAE中以多器官功能障碍为表现的患者,其病死率约为70%。这对患者家庭和其看护人来说,带来的心理伤害则无法估算。
在危重病患者中,SAE通常会出现镇静催眠和神经肌肉阻滞的相关症状。脑电图(electroencephalography,EEG)和体感诱发电位技术常用于患者床旁辅助诊断,而一些评分工具,如格拉斯哥评分、CAM-ICU和里士满躁动镇静评分等,则在测量患者临床动态变化方面具有较好的可行性和价值。SAE患者的头颅MRI可发生大脑半球半卵圆中心脑白质病变、多次缺血性卒中和脑容积减少等征象。虽然头颅MRI诊断SAE可能不一定能够及时获得阳性的发现,但在50%的病例中,甚至在症状出现之前就可能存在EEG异常表现。在EEG上,θ波的“减慢”与轻度SAE相关,重度病例表现为δ波、三相波和爆发抑制。
一、SAE的病理变化
脓毒症脑病的发病机制复杂且未完全阐明。中枢微循环障碍、低灌注和外周脏器组织坏死导致全身炎症状态,其中白细胞——特别是小胶质细胞的活化、溶酶体胞吞、细胞因子释放和自由基生成。脓毒症动物模型显示一氧化氮可介导海马和大脑皮质氧化应激损伤,而抗氧化剂和神经保护因子如热休克蛋白和超氧化物歧化酶减少。在神经介导的炎性反射中,神经传递沿迷走神经从外周传递到脑干孤束核。神经胶质细胞和CNS神经元依赖氨基酸、葡萄糖和氧代谢产生ATP并进行神经传递,而脓毒症的分解代谢状况破坏其生物能量来源和平衡。目前,对SAE发病机制的理解是多因素的,涉及细胞因子效应、线粒体功能障碍、神经递质改变以及缺氧导致的氧化损伤、缺血以及细胞死亡。病理改变包括播散性脑微脓肿性病变、多病灶的坏死性脑白质病和功能性脑血管密度降低。
二、血-脑屏障的结构和功能
BBB是保护大脑与循环和免疫系统接口的物理屏障。这种中枢神经系统和脊髓与外周器官的分离最早是在1885年Ehrlich注射某种“活性”染料时第一次观察到的。
Lewandowsky于1900年将其命名为“血-脑屏障”(BBB)。Reese和Karnovsky在一项具有里程碑意义的体外研究中发现,辣根过氧化物酶循环能通过外周血管系统,但没有通过大脑内皮细胞进入中枢神经系统。BBB的主要成分是脑微血管内皮细胞(BMVEC),其位于血管周细胞、星形胶质细胞足突和大胶质细胞的附近。因为离CNS神经元只有8~20μm的短距离,因此各类细胞间的扩散很容易实现。BMVEC通过由细胞外基质蛋白组成的基膜(包括胶原蛋白、弹性蛋白、纤连蛋白、层粘连蛋白和蛋白聚糖)连接到周细胞和星形胶质细胞。一些小管和窗孔样结构在这些细胞之间形成间隔,这样的结构限制了液体的流动,并进一步防止毛细血管泄漏。选择素、整合素和免疫球蛋白家族的跨膜蛋白组成的黏着斑将BMVEC固定在基膜上。其中,整合素参与血管生成和维持血管完整性,而黏着斑将机械力从细胞骨架传递到周围的黏附和收缩结构。构架维持由细胞黏附分子(CAMs)和紧密连接提供,细胞黏附分子在基膜顶端表达,紧密连接相邻的内皮细胞,限制细胞间扩散和细胞旁通透性。
BBB主要成分是跨膜蛋白,如连接黏附分子、紧密连接蛋白和闭合带蛋白1~3(闭合蛋白的主要作用为连接肌动蛋白细胞骨架,并介导细胞-细胞相互作用)。这种高达1500~2000Ω/cm2的高电阻的紧密连接分子结构限制了胞内和跨细胞移动。邻近的星形胶质细胞和小胶质细胞通过释放细胞因子来调整紧密连接的结构。此外,星形胶质细胞通过Wnt/B-连环蛋白介导的信号转导对屏障生成产生局部调节作用。周细胞或血管平滑肌细胞包绕BBB毛细血管内皮,并具有构成和调节功能,它们构成基膜的蛋白质,特别是蛋白聚糖和薄层蛋白。在空间上,它们覆盖其表面近三分之一,并为屏障提供结构完整性。细胞外肽酶和核酸酶可分解蛋白质和ATP,而单胺氧化酶和细胞色素p450在细胞内起作用,使神经毒性化合物失活。这些相邻的细胞和结构元件共同在细胞外基质环境中以相互协调的方式作为神经血管单元的一部分,并发挥相应作用。
三、跨血-脑屏障的物质运输
BBB维持中枢神经系统内环境稳定的能力是由其控制离子、小分子、免疫细胞、细胞因子、趋化因子和外源化合物转运的方式、速率和调节的能力决定的。在生理条件下,营养物质和必需分子可较为容易的进入中枢神经系统,而细胞代谢废物、毒素、神经活性物质和病原体则被抵挡在外。离子、水和小分子物质通过细胞旁扩散穿过BBB,而较大的亲水化合物如氨基酸和葡萄糖需要特定的转运系统进行跨细胞迁移。BBB在应对炎症和感染性刺激方面具有四项基本和独立的功能。它的屏障结构与应答、转运和分泌功能共存,共同维持分子转运稳态。“吸附”和“受体介导的内吞”这两种方式是蛋白质通过BBB和利用囊泡主要的主动转运手段。特异性转运蛋白存在于质膜上,如GLUT-1和ATP结合转运蛋白(ABC)。p-GP是一种ABC转运蛋白,是一种外排性泵分子,参与药物的输送、解毒并参与耐药机制。在内皮细胞膜上,胆固醇和鞘脂形成的板块称为“脂质筏”,其是经由细胞内胆固醇结合窝蛋白生成的。这个过程开始于形成60~80nm的称为洞穴状内陷的结构,然后形成“网格蛋白包被的小凹”。在BBB,小凹参与受体易位、囊泡转运和细胞信号转导(如IL-1β依赖性NF-kB活化)。
四、补体、细胞因子与血-脑屏障破坏的分子机制
补体系统的激活对于固有免疫系统抵御感染至关重要,并且这一点在SAE的炎症和神经元功能障碍的机制中已经得到了证明。一旦补体级联反应被内毒素激活,C5a便会作用于大脑内皮、小胶质细胞和脑实质的神经元。在模拟缺血再灌注损伤的研究中发现,C3a和C5a作为白细胞趋化因子发挥作用。随后内皮细胞和小胶质细胞被激活,分泌TNF-α和IL-1β,并最终导致ROS产生、脑水肿和严重的CNS神经元损伤(图1)。

在BBB的CNS这一侧,TNF-α可介导不同途径的神经元损伤,包括NO通路、细胞凋亡蛋白酶通路的细胞凋亡,并最终导致脑水肿。
在创伤、缺血、脓毒症等神经炎症状态下,细胞因子在神经损伤的发病机制中起关键作用。Semmler等发现,脑组织匀浆中趋化因子MCP-1、细胞因子IL-1β、TGF-β和TNF-α均与诱导型一氧化氮(iNOS)表达一起上调。这些研究结果可以解释脓毒症对CNS的影响,iNOS介导少突胶质细胞损伤,同时生成的NO的通过BAX-和p-53依赖途径诱导星形胶质细胞凋亡。
脓毒症时,IL-1β和TNF-α均升高,细胞因子间相互作用,并通过影响紧密连接稳定性和内皮通透性而影响BBB。脑内皮细胞上表达被称为Toll样受体(TLR)的模式识别受体,这些受体是对病原体和相关蛋白包括LPS的反应中的必要介质。在血-脑屏障内皮细胞中,TNF-α介导TLR2上调,TLR2/6的配体与其结合会介导紧密连接断裂。TNF-α可导致肌动蛋白解聚和内皮细胞骨架中细胞间隙产生。秦等发现,腹腔注射LPS后中枢内的TNF-α独立于循环内的TNF-α,这与黑质、海马和皮质中的小胶质活化同时发生,其他炎症介质如MCP-1、IL-1β和NF-kB也有所上调。O’Caroll等研究发现,在培养的内皮细胞中,TNF-α和IL-1β均可增加白细胞黏附分子的表达,包括ICAM-1和V-CAM-1、趋化因子MCP-1(CCL-2)和 RANTES(CCL-5),导致屏障功能障碍和跨内皮细胞的电阻降低。研究还发现TNF-α通过激活蛋白激酶-6增加屏障通透性,引起VE-钙黏蛋白内化的细胞-细胞相互作用。
在BBB中,TNF-α直接作用于内皮细胞,也可在没有屏障的区域扩散到脑实质中(如脑室周围器官)。TNF受体1,也称为p55,在脑中含量丰富,在星形胶质细胞中结构性表达。在炎症状态下,包括脓毒症过程中,TNF-α与TNF-R1结合。这种相互作用由细胞内TNF受体相关死亡结构域介导,该结构域招募TNF受体相关因子2。TNR受体相关因子 2(TNR-receptor associated factor 2,TRAF-2)是介导抗凋亡信号的TNF受体的衔接蛋白。
夏等发现,抗凋亡的信号转导由神经鞘脂介导,并涉及 TNF-α、TNF-R和TRAF-2之间的相互作用(图2)。同时,被称为小凹的膜内陷结构可促进TNF穿过内皮细胞。胞质内信号通过RhoA和RAC介导,最终通过NF-kB介导转录,产生抗凋亡和促凋亡蛋白。凋亡通过激活凋亡相关蛋白酶介导的死亡受体途径发生,其中活化的小胶质细胞是其中的参与者。Alexander等在SAE小鼠模型中阐明了LPS、TNF-α、TNF-R之间的相互作用及其对SAE的影响。研究者们发现,与相同条件下的TNFR-1双敲除小鼠相比,LPS可导致TNFR-1途径依赖性的星形胶质细胞活化和AQP通道介导的脑水肿增加。

图中描述了LPS和TNF-α对血-脑屏障的作用和导致神经认知功能障碍的病理生理机制。(A)LPS与其配体Toll样受体4(TLR-4)在脑微血管内皮细胞表面结合,同时TNF-α与TNF-α受体结合。(B)BBB完整性丧失,通常无法进入的分子现在能够迁移到CNS神经元间隙,包括TNF-α。TNF-α与胶质细胞相互作用,导致神经元损伤、凋亡、少突胶质细胞减少和反应性星形胶质细胞增生。海马神经元损伤和凋亡是谵妄和长期神经认知障碍的可能机制。(C)在BBB内皮细胞的外侧,TRAF-2、TNF-α和Sphk-1形成复合物,该复合物催化胞质中神经鞘氨醇-1-磷酸(S-1-P)的形成。S-1-P通过自分泌功能离开细胞,作用于内皮表面的S-1-P受体。S-1-P受体的跨膜区域发生构象变化,并激活GTPases RhoA和RAC,导致肌动蛋白和肌球蛋白的重新排列和BBB完整性重建。(D)S-1-P还对CNS神经元发挥旁分泌活性,帮助细胞存活和阻止凋亡发生。
五、海马在SAE中的重要作用及与阿尔茨海默病(AD)病理改变的相关性
海马是参与炎症介质和突触功能改变的关键部位,并在SAE的发病机制中发挥关键作用(图3)。给予LPS处理与学习记忆能力损伤密切相关。Lynch等发现LPS直接抑制海马齿状回和长时程增强,同时Imamura等发现,这与IL-1β呈平行变化。胆碱能神经传递与认知直接相关,抑制胆碱能神经传递与谵妄情况加重相关。此外,血-脑屏障破坏与海马损伤、认知障碍有关。Hellstrom等对海马CA1区锥体神经元暴露于LPS 7天后进一步研究,发现LPS神经毒性模型中突触功能障碍的机制,并发现暴露组具有较低的细胞膜电阻抗、较高的动作电位阈值和较慢的动作电位放电频率。

血-脑屏障(BBB)在脓毒症相关脑病的神经认知损伤机制中起着不可或缺的作用。脂多糖通过紧密连接的结构改变和跨内皮转运的改变降低屏障功能完整性。鞘氨醇-1-磷酸(S-1-P)或类似物被提出来增强屏障完整性,潜在地减轻脓毒症相关脑病的神经认知后遗症。
六、鞘脂作为SAE治疗靶点的潜力
脓毒症治疗的主要措施包括液体治疗和抗生素,早期给药可预防终末器官损伤。最近的研究探索了静脉注射免疫球蛋白(intravenous immunoglobulin,IVIG)、镁、类固醇、高剂量维生素和单克隆抗体。有研究显示,应用IVIG后,与对照组相比,接受盲肠结扎穿孔的实验鼠死亡率下降,BBB渗透性降低。而SAE的神经精神症状仍然难以治疗,目前仅局限于对谵妄症状的干预和护理。我们对脑中神经鞘脂信号的理解的进展为SAE治疗药物靶点的开发提供了新的途径。神经鞘脂是在多种器官系统的细胞膜中发现的生物活性脂质家族,它们在介导血管通透性、细胞信号转导、细胞存活和凋亡中必不可少。其中一种鞘脂——鞘氨醇 -1-磷酸(sphingosine-1-phosphate,S-1-P)已经被发现在血管生成和膜稳定性、免疫细胞运输以及细胞增殖、分化、存活和癌变中发挥不可或缺的作用。鞘氨醇是在鞘氨醇酶催化鞘磷脂产生神经酰胺后产生的,其由神经酰胺酶作用。鞘氨醇激酶-1(SphK)将鞘氨醇磷酸化形成S-1-P,SphK是一种42kDa的脂质激酶,在脑、心、肺和脾中含量较高。在内皮细胞中,S-1-P由活化的血小板释放,也是内皮差异识别基因家族编码的G蛋白偶联受体的配体,现在称为(S-1-P)1-5。S-1-P还充当第二信使,参与信号转导,例如在少突胶质细胞S-1-P是s1p 5受体上的配体。其下游效应包括钙调节、细胞增殖、迁移和抗凋亡。
鞘氨醇-1-磷酸在脓毒症诱导的内皮功能障碍中具有机制和治疗作用,并且在炎症状态下对星形胶质细胞、神经元和胶质细胞以及BBB内皮具有调节活性。Garcia等发现S-1-P可降低血管内皮通透性。S-1-P与受体结合并与Gi/α发生偶联后,通过p38 MAP激酶和ERK 1\2促进信号转导。Peng等研究发现,S-1-P在小鼠脓毒症模型中对内皮产生一定影响。他们行腹腔内注射LPS,然后在小鼠肺中测量标记染料和渗出蛋白,以及其他跨内皮细胞迁移标记物。研究观察到S-1-P及其类似物FTY-720均可显著减少血管渗漏和炎性细胞渗出。这项工作支持先前的研究结论,表明鞘氨醇磷酸酯可提高内皮稳定性。已证明S-1-P通过激活血小板中的鞘氨醇-正弦激酶磷酸化而大量产生,并充当鞘氨醇受体上的配体。随后,GTPases Rho和Rac与蛋白激酶C一起被激活,导致胞内钙的增加和肌动蛋白的转录导,从而发生细胞骨架的改变。肌动蛋白是屏障稳定性的关键介质,因此这一个过程较为重要。S-1-P及其类似物FTY-720在LPS诱导的炎症条件下,对内皮毛细血管具有重建活性(图2)。内皮完整性通过肌动蛋白环的形成和肌动蛋白结合蛋白的修饰得到加强。有研究证明,在LPS介导的炎症损伤过程中,抑制肺内皮脂质筏上的鞘磷脂合成酶(SMS),可抑制鞘磷脂信号转导,保护屏障内皮完整性。用SMS抑制剂D609处理后,可观察到细胞骨架重排,其表现为外周肌动蛋白增加,肌动蛋白和肌球蛋白相互作用形成皮质肌动蛋白环。
七、中枢神经系统中的神经鞘脂
脓毒症诱导的肺内皮改变可能适用于其他器官系统。鞘脂在BBB和细胞水平上对中枢神经系统发挥积极作用,并可与病理状态相关联。例如,在实验条件下,Cannon等研究显示鞘氨醇类似物FTY-720通过TNF/TNFR和鞘氨醇单一途径影响p-糖蛋白介导的BBB的药物摄取。在帕金森病模型中,Martinez等证明在黑质中,多巴胺能神经元对细胞凋亡蛋白酶介导的细胞毒性较为敏感,并且该途径也依赖于TNF和神经酰胺。他们的研究还显示,通过抑制鞘磷脂酶,细胞毒性作用减弱。另外,Psyzko等研究显示,S-1-P、SphK-1和FTY-720p改善PD模型中多巴胺能细胞的氧化应激反应。Grinkina等研究了鞘脂类信号在脓毒症引起的中枢神经系统炎症中的作用。研究使用SphK敲除小鼠,在SphK−/−属脑内注射LPS后,与野生型相比,观察到明显的病理变化,包括心室大小增加、脑白质疏松程度加重和脑白质间隙增加,这些结果共同提示脑白质丢失。SphK−/−组中枢神经系统常驻细胞出现显著功能障碍,表现为反应性小胶质细胞增生、GFAP过度表达(表明星形胶质细胞活化)和少突胶质细胞丢失。
FTY-720,也称为Fingolimod,目前被批准用于多发性硬化的治疗,其被充分证明是阻碍淋巴组织T细胞迁移的免疫调节剂。利用鞘脂治疗其他中枢神经系统疾病(包括SAE)具有巨大潜力。具体而言,作为鞘氨醇类似物,Fingolimod通过作用于S-1-P受体修饰内皮从而降低内皮通透性。Brinkmann等研究显示,S-1-P抑制VEGF介导的血管通透性,并且S-1-P和磷酸化的FTY-720都增强了内皮细胞-细胞连接组件。S-1-P受体在CNS中几乎普遍存在,存在于少突胶质细胞、星形胶质细胞和神经元上,作为正常神经功能执行和修复的关键介质。例如,S-1-P对少突胶质细胞产生效应可导致髓鞘再生,而FTY-720及其代谢产物FTY-720-P可减少皮质神经元兴奋毒性介导的细胞死亡。此外,Kanno等研究显示海马中S-1-P/Sph-K信号与突触的增强和记忆和学习任务改善相关。
与SAE相似,AD也表现为严重的认知功能障碍,其中神经炎症、脂质失调和斑块形成是疾病进展的可能机制。AD患者脑中神经酰胺水平显著增加,从而干扰了糖酵解,并促进氧化应激,最终导致A-β蛋白产生,提示鞘脂在发病机制中起关键作用。鞘脂在SAE和AD所致严重认知功能障碍中具有共同特征,最近的临床前研究成功将FTY-720在AD模型应用,这可作为SAE治疗的潜在靶点,海马是这两种疾病的主要病理学变化发生的位置。此外,BBB功能障碍与认知能力下降和脓毒性脑病有关,这一研究结果使其成为FTY-720的理想的应用靶标。一方面,AD患者大脑S-1-P含量相对减少,这意味着S-1-P激动剂可能在治疗中具有一定价值。基于神经酰胺水平与AD中鞘氨醇成反比的结论,以及FTY-720具有潜在神经保护的特性等这样一些结果,Asle-Rousta等发现这种复合物在啮齿动物AD模型中有效。与对照组相比,研究组长期应用FTY-720可显著消除A-β42诱导的海马CA1区神经元凋亡。Aytan等在转基因小鼠研究中显示,给予Fingolimod可降低淀粉样β斑块密度,抑制小胶质细胞活化,并显著降低海马星形胶质细胞增生。此外,Kolahdooz等研究两种不同的S-1-P激动剂FTY-720和SEW7821对神经炎症和LPS诱导的记忆损伤的作用。作者发现,FTY-720可显著降低LPS诱导的记忆缺陷,两种药物均恢复了LPS诱导的鞘磷脂代谢酶如SphK-1的变化。
总之,SAE和AD共同的病理生理学相关性,以及FTY-720及其类似物的成功应用,强化了S-1-P在治疗开发中的积极作用,而这一领域需要进行进一步深入研究。
八、结论
尽管对脓毒症脑病发生的分子机制有了一定深入了解,但仍是一个较为复杂、困惑的临床问题。SAE和以海马为病变中心的AD具有显著的相关性,为研究提供了一个共同机制框架,我们可以用它来探讨脓毒症中的认知功能障碍。利用我们对鞘脂、FTY-720或鞘脂类似化合物的知识,可能能够更好掌握稳定CNS和预防神经炎症损伤的关键,并可以预防SAE的长期后遗症,包括认知障碍、功能依赖和长期住院。
参考文献
1.Singer M,Deutschman CS,Seymour CW,et al. The third international consensus definitions for sepsis and septic shock(sepsis-3).JAMA,2016,315(8):801-810.
2.Adam N,Kandelman S,Mantz J,et al. Sepsis-induced brain dysfunction. Expert Rev Anti Infect Ther,2013,11(2):211-21.
3.Ebersoldt M,Sharshar T,Annane D. Sepsis-associated delirium. Intensive Care Med,2007,33(6):941-950.
4.Sonneville R,Verdonk F,Rauturier C,et al. Understanding brain dysfunction in sepsis. Ann Int Care,2013,3:15.
5.Papadopoulos MC,Davies DC,Moss RF,et al. Pathophysiology of septic encephalopathy:a review. Crit Care Med,2000,28(8):3019-3024.
6.Sprung CL,Peduzzi PN,Shatney CH,et al. Impact of encephalopathy on mortality in the sepsis syndrome. The veterans administration systemic sepsis cooperative study group. Crit Care Med,1990,18(8):801-806.
7.Eidelman LA,Putterman D,Putterman C,et al. The spectrum of septic encephalopathy. Definitions,etiologies,and mortalities. JAMA,1996,275(6):470-473.
8.Neufeld KJ,Thomas C. Delirium:definition,epidemiology,anddiagnosis. J Clin Neurophysiol,2013,30(5):438-442.
9.Gofton TE,Young GB. Sepsis-associated encephalopathy. Nat Rev Neurol,2012,8(10):557-566.
10.Barichello T,Fortunato JJ,Vitali AM,et al. Oxidative variables in the rat brain after sepsis induced by cecal ligation and perforation. Crit Care Med,2006,34(3):886-889.
11.Iwashyna TJ,Ely EW,Smith DM,et al. Long-term cognitive impairment and functional disability among survivors of severe sepsis. JAMA,2010,304(16):1787Y1794.
12.Iwashyna TJ,Cooke CR,Wunsch H,et al. Population burden of long-term survivorship after severe sepsis in older Americans. J Am Geriatr Soc,2012,60(6):1070-1077.
13.Iacobone E,Bailly-Salin J,Polito A,et al. Sepsis-associated encephalopathy and its differential diagnosis. Crit Care Med,2009,37(10):S331-336.
14.Hosokawa K,Gaspard N,Su F,et al. Clinical neurophysiological assessment of sepsis-associated brain dysfunction:a sys- tematic review. Crit Care,2014,18(6):674.
15.Young GB,Bolton CF,Archibald YM,et al. The electroencephalogram in sepsis-associated encephalopathy. J Clin Neurophysiol,1992,9(1):145-152.
16.Tighe D,Moss R,Boghossian S,et al. Multiorgan damage resulting from experimental faecal peritonitis. Clin Sci,1989,76:269-276.
17.Bozza FA,D’Avila JC,Ritter C,et al. Bioenergetics,mitochondrial dysfunction,and oxidative stress in the pathophysiology of septic encephalopathy. Shock,2013,39(Suppl 1):10-16.
18.Jackson AC,Gilbert J,Young GB,et al. The encephalopathy of sepsis. Can J Neurol Sci,1985,12:303-307.
19.Sharshar T,Polito A,Checinski A,et al. Sepsis-associated encephalopathy everything starts at a microlevel. Crit Care,2010,14(5):1999.
20.Sharshar T,Gray F,Poron F,et al. Multifocal necrotizing leukoencephalopathy in septic shock. Crit Care Med,2002,30:2371-2375.
21.Taccone FS,Su F,De Deyne C,et al. Sepsis is associated with altered cerebral microcirculation and tissue hypoxia in experimental peritonitis. Crit Care Med,2014,42(2):e114-122.
22.Banks WA,Erickson M. The blood-brain barrier and immune function and dysfunction. Neurobiol Dis,2010,37:26-32.
23.Reese TS,Karnovsky MJ. Fine structural localization of a blood-brain barrier to exogenous peroxidase. J Cell Biol,1967,34:207-17.
24.Tsao N,Hsu HP,Wu CM,et al. Tumour necrosis factor-α causes an increase in blood-brain barrier permeability during sepsis. J Med Microbiol,2001,50:812-821.
25.Cardoso F,Brites D,Brito M. Looking at the blood-brain barrier:molecular anatomy and possible investigation approaches. Brain Res Rev,2010,64(2):328-363.
26.Nico B,Ribatti D. Morphofunctional aspects of the bloodbrain barrier. Curr Drug Metab,2012,13(1):50-60.
27.Pardridge WM. Drug transport across the blood-brain barrier. J Cereb Blood Flow Metab,2012,32(11):1959-1972.
28.Banks WA. The blood-brain barrier in neuroimmunology:tales of separation and assimilation. Brain Behav Immun,2014,44:1-8.
29.Hawkins BT,Davis TP. The blood-brain barrier/neurovascular unit in health and disease. Pharmacol Rev,2005,57(2):173-85.
30.Pardridge WM. Blood-brain barrier biology and methodology. J Neurovirol,1999,5(6):556-556
31.Ballabh P,Braun A,Nedergaard M. The blood-brain barrier:an overview:structure,regulation,and clinical implications. Neurobiol Dis,2004,16:1-13.
32.Abbott NJ,Friedman A. Overview and introduction:the blood-brain barrier in health and disease. Epilepsia,2012,53(6):1-6.
33.Abbott NJ,Rönnbäck L,Hansson E. Astrocyte-endothelial interactions at the blood-brain barrier. Nat Rev Neurosci,2006,7(1):41-53.
34.Persidksy Y,Ramirez S,Haorah J,et al. Blood-brain barrier:structural components and function under physiologic and pathologic conditions. J Neuroimm Pharm,2006,1(3):223-236.
35.Wolburg H,Lippoldt A. Tight junctions of the blood-brain barrier:devel-opment,composition and regulation. Vascul Pharmacol,2002,38:323-337.
36.Yuan H,Gaber MW,McColgan T,et al. Radiation-induced permeability and leukocyte adhesion in the rat blood- brain barrier:modulation with anti-ICAM-1 antibodies. Brain Res,2003,969(1-2):59-69.
37.Abbott NJ,Patabendige AA,Dolman DE,et al. Structure and function of the blood-brain barrier. Neurobiol Dis,2010,37(1):13-25.
38.Jin L,Nation RL,Li J,et al. Species-dependent bloodbrain barrier disruption of lipopolysaccharide:amelioration by colistin in vitro and in vivo. Antimicrob Agents Chemother,2013,57(9):4336-4342.
39.Butt AM,Jones HC,Abbott NJ. Electrical resistance across the blood-brain barrier in anaesthetized rats:a developmental study. Physiol,1990,429:47-62.
40.de Boer AG,Gaillard PJ. Blood-brain barrier dysfunction and recovery. Neural Transm,2006,113(4):455-62.
41.Liebner S,Corada M,Bangsow T,et al. Wnt/beta-catenin signaling controls development of the blood-brain barrier. Cell Biol,2008,183:409-417.
42.Obermeier B,Daneman R,Ransohoff R. Development,maintenance and disruption of the blood-brain barrier. Nat Med,2013,19(12):1584-1596.
43.Kim JA,Tran ND,Li Z,et al. Brain endothelial hemostasis regulation by pericytes. Cereb Blood Flow Metab,2006,26(2):209-217.
44.Lai CH,Kuo KH,Leo JM. Critical role of actin in modulating BBB permeability. Brain Res Rev,2005,50:7-13.
45.El-Bacha RS,Minn A. Drug metabolizing enzymes in cerebrovascular endothelial cells afford a metabolic protection to the brain. Cell Mol Biol,1999,45:15-23.
46.Bauer B,Hartz AM,Miller DS. Tumor necrosis factor alpha and endothelin-1 increase P-glycoprotein expression and transport activity at the blood-brain barrier. Mol Pharmacol,2007,71(3):667-675.
47.Cannon R,Peart J,Hawkins B,et al. Targeting bloodbrain barrier sphingolipid signaling reduces basal P-glycoprotein activity and improves drug delivery to the brain. Proc Natl Acad Sci U S A,2012,109(39):15930-15935.
48.Pan W,Stone KP,Hsuchou H,et al. Cytokine signaling modulates blood-brain barrier function. CurrPharm Des,2011,17(33):3729-3740.
49.D’Alessio A,Al-Lamki RS,Bradley JR,et al. Caveolae participate in tumor necrosis factor receptor 1 signaling and internalization in a human endothelial cell line. Am J Pathol,2005,166(4):1273-1282.
50.H,Wu C-H,Shackleford GM,et al. Lipid raft/caveolae signaling is required for Cryptococcus neoformans invasion into human brain microvascular endothelial cells. J Biomed Sci,2012,19(1):19.
51.Parton R,Simons K. The multiple faces of caveolae. Nat Rev Mol Cell Biol,2007,8:185-194.
52.Nag S,Manias JL,Stewart DJ. Expression of endothelial phosphorylated caveolin-1 is increased in brain injury. Neuropathol Appl Neurobiol,2009,35(4):417-426.
53.Alexander J,Jacob A,Cunningham P,et al. TNF is a key mediator of septic encephalopathy acting through its receptor,TNF receptor-1. Neurochem Int,2008,52(3):447-456.
54.Jacob A,Brorson JR,Alexander JJ. Septic encephalopathy:inflammation in man and mouse. Neurochem Int,2011,58(4):472-476.
55.Jacob A,Hack B,Chen P,et al. C5a/CD88 signaling alters blood-brain barrier integrity in lupus through nuclear factor-κB. JNeurochem,2011,119(5):1041-1051.
56.Jacob A,Alexander JJ. Complement and blood-brain barrier integrity. Mol Immunol,2014,61(2):149-152.
57.Ducruet AF,Zacharia BE,Hickman ZL,et al. The complement cascade as a therapeutic target in intracerebral hemorrhage. Exp Neurol,2009,219(2):398-403.
58.Aronowski J,Hall CE. New horizons for primary intracerebral hemorrhage treatment:experiencefrompreclinicalstudies. Neurol Res,2005,27(3):268-279.
59.Semmler A,Hermann S,Mormann F,et al. Sepsis causes neuroinflammation and concomitant decrease in cerebral metabolism. J Neuroinflamm,2008,5:38.
60.Li J,Baud O,Vartanian T,et al. Peroxynitrite generated by inducible nitric oxide synthase and NADPH oxidase mediates microglial tox- icity to oligodendrocytes. Proc Natl Acad Sci U S A,2005,102(28):9936-9941.
61.Yung HW,Bal-Price AK,Brown GC,et al. Nitric oxideinduced cell death of cerebrocortical murine astrocytes is mediated through p53-and Bax-dependent pathways. J Neurochem,2004,89:812-821.
62.Cannon JG,Tompkins RG,Gelfand JA,et al. Circulating interleukin-1 and tumor necrosis factor in septic shock and experimental endotoxin fever. J Infect Dis,1990,161:79-84.
63.Nadeau S,Rivest S. Effects of circulating tumor necrosis factor on the neuronal activity and expression of the genes encoding the tumor necrosis factor receptors(P55 and P75)in the rat brain:a view from the blood-brain barrier. Neuroscience,1999,93(4):1449-1464.
64.Andreasen A,Krabbe K,Krogh-Madsen R,et al. Human endotoxemia as model of systemic inflammation. Curr Med Chem,2008,15:1697-1705.
65.Bauer HC,Krizbai IA,Traweger A. “You shall not pass”tight junctions of the blood brain barrier. Front Neurosci,2014,8:392.
66.Nagyoszi P,Wilhelm I,Farkas AE,et al. Expression and regulation of toll-like receptors in cerebral endothelial cells. Neurochem Int,2010,57(5):556-564.
67.Goldblum SE,Ding X,Campbell-Washington J. TNF-α induces endothelial cell F-actin depolymerization,new actin synthesis,and barrier dysfunction. Am J Physiol,1993,264:894-905.
68.Qin L,Wu X,Block ML,et al. Systemic LPS causes chronic neuroinflammation and progressive neurodegeneration. Glia,2007,55(5):453-462.
69.O’Carroll SJ,Kho DT,Wiltshire R,et al. Pro-inflammatory TNF-α and IL-1β differentially regulate the inflammatory phenotype of brain microvascular endothelial cells. J Neuroinflammation,2015,12:131.
70.Haines RJ,Beard RS Jr,Wu MH. Protein tyrosine kinase 6 mediates TNFα- induced endothelial barrier dysfunction. Biochem Biophys Res Commun,2015,456(2):190-196.
71.Dopp JM,Mackenzie-Graham A Jr,Otero GC,Merrill JE. Differential expression,cytokine modulation,and specific functions of type-1 and type-2 tumor necrosis factor receptors in rat glia. Neuroimmunol,1997,75(1-2):104-12.
72.Xia P,Wang L,Moretti PA,et al. Sphingosine kinase interacts with TRAF2 and dissects tumor necrosis factoralpha signaling. Biol Chem,2002,277(10):7996-8003.
73.Cheng ZJ,Singh RD,Sharma DK,et al. Distinct mechanisms of clathrin-independent endocytosis have unique sphingolipid requirements. Mol Biol Cell,2006,17(7):3197-3210.
74.Hengartner MO. The biochemistry of apoptosis. Nature,2000,407:770-776.
75.Yuan J,Yankner BA. Apoptosis in the nervous system. Nature,2000,407:802-809.
76.Burguillos MA,Deierborg T,Kavanagh E,Pet al. Caspase signalling controls microglia activation and neurotoxicity. Nature,2011,472(7343):319-324.
77.Krabbe KS,Reichenberg A,Yirmiya R,et al. Low-dose endotoxemia and human neuropsychological functions. Brain Behav Immun,2005,19(5):453-460.
78.Czerniawski J,Guzowski JF. Acute neuroinflammation impairs context discrimination memory and disrupts pattern separation processes in hippocampus. J Neurosci,2014,34:1247-1280.
79.Hasegawa-Ishii S,Inaba M,Umegaki H,et al. Endotoxemia-induced cytokine-mediated responses of hippocampal astrocytes transmitted by cells of the brain-immune interface. Sci Rep,2016,6:25457.
80.Shaw KN,Commins S,O’Mara SM. Lipopolysaccharide causes deficits in spatial learning in the water maze but not in BDNF expression in the rat dentate gyrus. Behav Brain Res,2001,124(1):47-54.
81.Lynch AM,Walsh C,Delaney A,et al. Lipopolysaccharide-induced increase in signalling in hippocampus is abrogated by IL-10 - a role for IL-1 beta? J Neurochem,2004,88(3):635-646.
82.Imamura Y,Wang H,Matsumoto N,et al. Interleukin-1β causes long-term potentiation deficiency in a mouse model of septic encephalopathy. Neuroscience,2011,187:63-69.
83.Semmler A,Frisch C,Debeir T,Ret al. Long-term cognitive impairment,neuronal loss and reduced cortical cholinergic innervation after recovery from sepsis in a rodent model. Exp Neurol,2007,204(2):733-740.
84.Montagne A,Barnes SR,Sweeney MD,et al. Bloodbrain barrier breakdown in the aging human hippocampus. Neuron,2015,85(2):296-302.
85.Costantino I. Dangerous leaks:blood-brain barrier woes in the aging hippocampus. Neuron,2015,85(2):231-233.
86.Hellstrom IC,Danik M,Luheshi GN,et al. Chronic LPS exposure produces changes in intrinsic membrane properties and a sustained IL-beta- dependent increase in GABAergic inhibition in hippocampal CA1 pyramidal neurons. Hippocampus,2005,15(5):656-664.
87.Marik PE,Khangoora V,Rivera R,et al. Hydrocortisone,vitamin C and thiamine for the treatment of severe sepsis and septic shock:a retrospective before-after study. Chest,2016,151(6):1229-1238.
88.Esen F,Senturk E,Ozcan PE,et al. Intravenous immunoglobulins prevent the breakdown of the blood-brain barrier in exper- imentally induced sepsis. Crit Care Med,2012,40(4):1214-1220.
89.Bryan L,Kordula T,Spiegel S,et al. Regulation and function of sphingosine kinases in the brain. Biochim Biophys Acta,2008,1781(9):459-466.
90.Okada T,Kajimoto T,Jahangeer S,et al. Sphingosine kinase/sphingosine 1-phosphate signalling in central nervous system. Cell Signal,2009,21(1):7-13.
91.Grin’kina NM,Karnabi EE,Damania D,et al. Sphingosine kinase 1 deficiency exacerbates LPS-induced neuroinflammation. PLoS One,2012,7(5):e36475.
92.Kono M,Allende ML,Proia RL. Sphingosine-1-phosphate regulation of mammalian development. Biochim Biophys Acta,2008,1781(9):435-441.
93.Pyne S,Pyne N. Sphingosine 1-phosphate signalling in mammalian cells. Biochem J,2000,349:385-402.
94.Mendelson K,Evan T,Hla T. Sphingosine-1-phosphate signaling. Development,2014,141:5-9.
95.Spiegel S,Milstien S. Sphingosine 1-phosphate,a key cell signaling molecule. Biol Chem,2002,277(29):25851-25854.
96.Hait NC,Oskeritzian CA,Paugh SW,et al. Sphingosine kinases,sphingosine 1-phosphate,apoptosis and diseases. Biochim Biophys Acta,2006,1758:2016-2026.
97.Peng X,Hassoun P,Sammani S,et al. Protective effects of sphingosine-1-phosphate in murine endotoxin-induced inflammatory lung injury. Am J Respir Crit Care Med,2004,169(11):1245-1251.
98.Schaphorst KL,Chiang E,Jacobs KN,Zaiman A,Natarajan V,Wigley F,et al. Role of sphingosine-1 phosphate in the enhancement of endothelial barrier Integrity by platelet-released products. Am J Physiol Lung Cell Mol Physiol,2003,285(1):L258-267.
99.Pitson S,Moretti P,Zebol J,Lynn H,Xia P,Vadas M.Activation of sphin- gosine kinase 1 by ERK1/2-mediated phosphorylation. EMBO,2003,22(20):5491-500.
100.Garcia JG,Liu F,Verin AD,Birukova A,Dechert MA,Gerthoffer WT,et al. Sphingosine 1-phosphate promotes endothelial cell barrier integrity by EDG- dependent cytoskeletal rearrangement. J Clin Invest,2001,108(5):689-701.
101.Buccoliero R,Futerman AH. The roles of ceramide and complex sphingolipids in neuronal cell function. Pharmacol Res,2003,47(5):409-419.
102.Wadgaonkar R,Patel V,Grinkina N,Romano C,Liu J,Zhao Y,et al. Differential regulation of sphingosine kinases 1 and 2 in lung injury. Am J Physiol Lung Cell Mol Physiol,2009,296(4):L603-613.
103.Zheng DM,Kitamura T,Ikejima K,Enomoto N,Yamashina S,Suzuki S,et al. Sphingosine 1-phosphate protects rat liver sinusoidal endothelial cells from ethanol-induced apoptosis:role of intracellular calcium and nitric oxide. Hepatology,2006,44(5):1278-1287.
104.Chun J,Hartung HP. Mechanism of action of oral fingolimod(FTY720)in multiple sclerosis. Clin Neuropharmacol,2010,33:91.
105.Tauseef M,Kini V,Knezevic N,et al. Activation of sphingosine kinase-1 reverses the increase in lung vascular per- meability through sphingosine-1-phosphate receptor signaling in endothe- lial cells. Circ Res,2008,103(10):1164-1172.
106.Shikata Y,Birukov KG,Garcia JG. S1P induces FA remodeling in human pulmonary endothelial cells:role of Rac,GIT1,FAK,and paxillin. J Appl Physiol,1985,94(3):1193-1203.
107.Shasby DM,Shasby S,Sullivan JM,et al. Role of endothelial cell cyto- skeleton in control of endothelial permeability. Circ Res,1982,51:657-661.
108.Natarajan V,Dudek SM,Jacobson JR,et al. Sphingosine-1-phosphate,FTY720,and sphingosine-1-phosphate receptors in the pathobiology of acute lung injury. Am J Respir Cell Mol Biol,2013,49(1):6-17.
109.Anjum F,Joshi K,Grinkina N,et al. Role of sphingomyelin synthesis in pulmonary endothelial cell cytoskeletal activation and endotoxin-induced lung injury. Am J Respir Cell Mol Biol,2012,47(1):94-103.
110.Gowda S,Yeang C,Wadgaonkar S,et al. Sphingomyelin synthase 2(SMS2)deficiency attenuates LPS-induced lung injury. Am J Physiol Lung Cell Mol Physiol,2011,300(3):L430-440.
111.Martinez TN,Chen X,Bandyopadhyay S,et al. Ceramide sphingolipid signaling mediates tumor necrosis factor(TNF)-dependent toxicity via caspase signaling in dopaminergic neurons. Mol Neurodegener,2012,13(7):45.
112.Pyszko J,Strosznaider J. Sphingosine kinase 1 and sphingosine-1-phosphate in oxidative stress evoked by 1-methyl-4-phenylpyridinium(MPP +)in human dopaminergic neuronal cells. Mol Neurobiol,2014,50(1):38-48.
113.O’Sullivan M. Leukoaraiosis. Pract Neurol,2008,8(1):26-38.
114.Gholamnezhadjafari R,Falak R,Tajik N,et al. Effect of FTY720(fingolimod)on graft survival in renal transplant recipients:a systematic review protocol. BMJ Open,2016,6(4):e010114.
115.Sanchez T,Estrada-Hernandez T,Paik JH,et al. Phosphorylation and action of the immunomodulator FTY720 inhibits vascular endothelial cell growth factor-induced vascular permeability. J Biol Chem,2003,278:47281-47290.
116.Brinkmann V,Davis MD,Heise CE,et al. The immune modulator fty720 targets sphingosine 1-phosphate receptors. Biol Chem,2002,277:21453-21457.
117.Foster CA,Howard LM,Schweitzer A,et al. Brain penetration of the oral immunomodulatory drug FTY720 and its phosphorylation in the central nervous system during experimental autoim- mune encephalomyelitis:consequences for mode of action in multiple scle- rosis. J Pharmacol Exp Ther,2007,323:469-475.
118.O’Sullivan S,Dev KK. Sphingosine-1-phosphate receptor therapies:advances inclinicaltrialsfor CNS-relateddiseases. Neuropharmacology,2017,113(Pt B):597-607.
119.Groves A,Kihara Y,Chun J. Fingolimod:direct CNS effects of sphingosine 1-phosphate(S1P)receptor modulation and implications in multiple sclero-sis therapy. J Neurol Sci,2013,328(1-2):9-18.
120.Miron VE,Jung CG,Kim HJ,Kennedy TE,Soliven B,Antel JP. FTY720 modulates human oligodendrocyte progenitor process extension and survival. Ann Neurol,2008,63(1):61-71.
121.Di Menna L,Molinaro G,Di Nuzzo L,et al. Fingolimod protects cultured cortical neurons against excitotoxic death. Pharmacol Res,2013,67(1):1-9.
122.Kanno T,Nishizaki T,Proia RL,et al. Regulation of synaptic strength by sphingosine 1-phosphate in the hippocampus. Neuroscience,2010,171(4):973-980.
123.Asle-Rousta M,Kolahdooz Z,Oryan S,et al. FTY720(fingolimod)attenuates beta-amyloid peptide(Aβ42)-induced impairment of spatial learning and memory in rats. Mol Neurosci,2013,50(3):524-532.
124.Filippov V,Song MA,Zhang K,et al. Increased ceramide in brains with Alzheimer’s and other neurodegenerative diseases. Alzheimers Dis,2012,29(3):537-547.
125.Asle-Rousta M,Kolahdooz Z,Dargahi L,et al. Prominence of central sphingosine-1-phosphate receptor-1 in attenuating aβ-induced injury by fingolimod. Mol Neurosci,2014,54(4):698-703.
126.Davies DC. Blood-brainbarrierbreakdowninsepticencephalopathyandbrain tumours. Anat,2002,200:639-646.
127.Kolahdooz Z,Nasoohi S,Asle-Rousta M,et al. Sphingosin-1-phosphate receptor 1:a potential target to inhibit neuroinflam- mation and restore the sphingosin-1-phosphate metabolism. Can J Neurol Sci,2015,42(3):195-202.
128.He X,Huang Y,Li B,et al. Deregulation of sphingolipid metabolism in Alzheimer’s disease. Neurobiol Aging,2010,31(3):398-408.
129.Aytan N,Choi JK,Carreras I,et al. Fingolimod modulates multiple neuroinflammatory markers in a mouse model of Alzheimer’s disease. Sci Rep,2016,6:24939.